Quandela’s innovative photonic architecture brings us to fault-tolerant quantum computing closer than ever
Fault tolerance represents a critical milestone in quantum computing. No matter the architecture you explore, current quantum computers are noisy, and one of the most challenging goals of quantum technologies involves performing reliable and useful tasks despite the noise affecting them.
During the last decades, engineers have developed a range of quantum error-correcting codes. The effective implementation of these codes demands thorough adaptation to the unique characteristics of each hardware and its corresponding noise model. It is this combination of error-correcting codes and hardware that will create a successful fault-tolerant quantum computer.
Quandela has developed a novel, robust method that leverages synergies between matter-based and photon-based quantum approaches to bring fault-tolerant quantum computing closer than ever. This new architecture is called Spin-Optical Quantum Computing (SPOQC –yes, just like Mr. Spock). This architecture is designed to be compatible with most state-of-the-art quantum error-correcting codes. Let’s dive deep into how it works.
Spin-Optical Quantum Computing Architecture
The SPOQC architecture is designed for single-photon emitters, devices capable of emitting one photon at a time. With these single photons, we usually create our qubits to carry information and perform operations on our quantum computers. A single-photon emitter can be physically created in many ways; however, the most efficient and reliable approach involves using quantum dots. A quantum dot is a tiny semiconductor device, only a few nanometers in size, that behaves as an artificial atom. When we send a laser pulse on the quantum dot, an electron can be excited to a higher state of energy, just like an atom. When the excited electron drops back into a lower energy state, it releases energy as light—a single photon.
Let’s focus on the interaction between the excited electron in the quantum dot and the photon it emits upon decay. Electrons have a few properties that make them suitable to represent a qubit. The SPOQC architecture leverages a quantum property of electrons called spin. Spin is an intrinsic property of subatomic particles that can be observed when a beam of atoms goes through an inhomogeneous magnetic field. For electrons, the spin can take two values that physicists represent with arrows up and down (↑, ↓). For the spin to encode a quantum bit (qubit), these values can exist in superposition of the two states. Now, in photonics, we also pick a property of the photons to represent a qubit, for instance, its polarization or the path they take in space while traveling in a circuit. The SPOQC architecture is designed for the excited electron in the quantum dot to have its spin state entangled with the photon it is emitting upon decay to a lower energy level. The photon thus encodes a qubit that we denote the spin-entangled photonic qubit (or entangled photon for short) as its state is entangled with the spin qubit of the quantum emitter that generated it.
But what does all this have to do with error corrections? Let’s have a look.
The SPOQC architecture and error corrections
Error-correcting codes can be represented by a special kind of graph called the Tanner graph. A graph has nodes representing entities and edges representing relations between entities. A Tanner graph has two distinct kinds of nodes, in this case: data qubit nodes and check qubit nodes. A data qubit is an information carrier qubit, and a check qubit allows us to detect errors. Implementing check qubits in the hardware is key: it allows efficient error detection and, therefore, an efficient way to correct them.
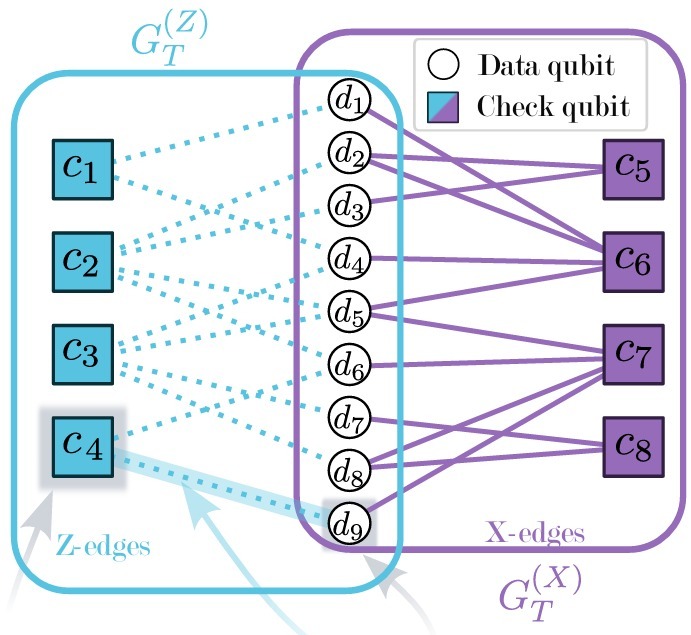
Implementing our SPOQC architecture, we mimic a Tanner graph in the hardware modularly, assembling circuits and connecting them repeatedly, like in a pattern, where optical fibers and other optical devices represent the edges of the graph. Each circuit has two single-photon emitters, where the entangled photons are created; in Quandela, we use quantum dots as single-photon emitters. One quantum dot emits an entangled photon related to the data qubit, and the other an entangled photon related to the check qubit. Both photons enter a repeat-unit-success gate or RUS gate. In the RUS gate, the photons undergo optical operations followed by detection. The detection process tells us if the optical operation was a failure or a success. If a failure is reported, the process is repeated. See the figure below.
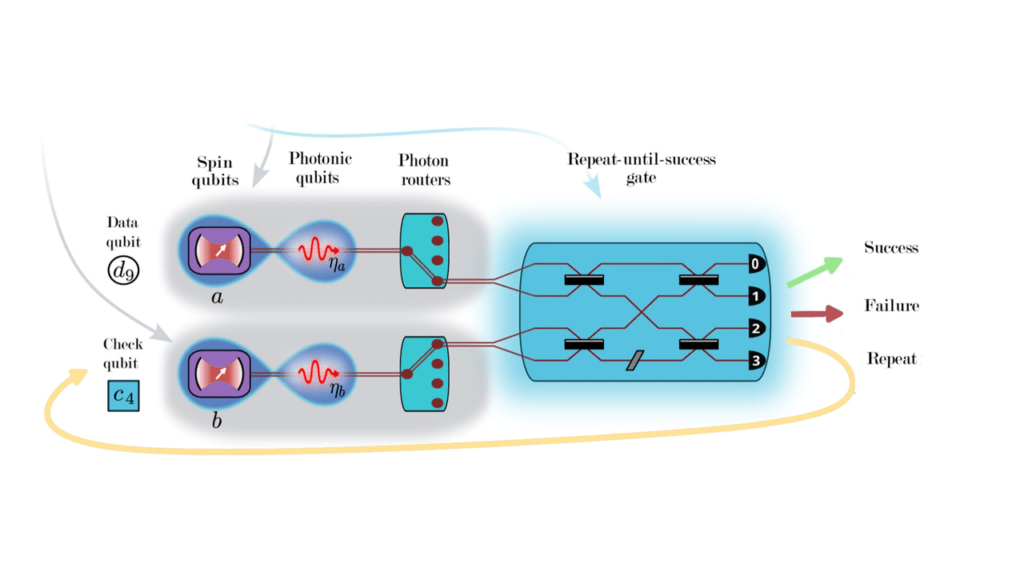
For an error-correcting code to be compatible with the hardware, it is crucial that the way in which the hardware modules are connected matches the Tanner graph of the error-correcting code to be used. Our SPOQC architecture allows us to create a layout suitable for implementing error-correcting methods that do not require direct interaction between the spin qubits. Instead, we use photons to mediate the two-qubit gates between them. In other words: our quantum dots send the entangled photons on a mission quest to help detect the errors while maintaining our spin qubits intact.
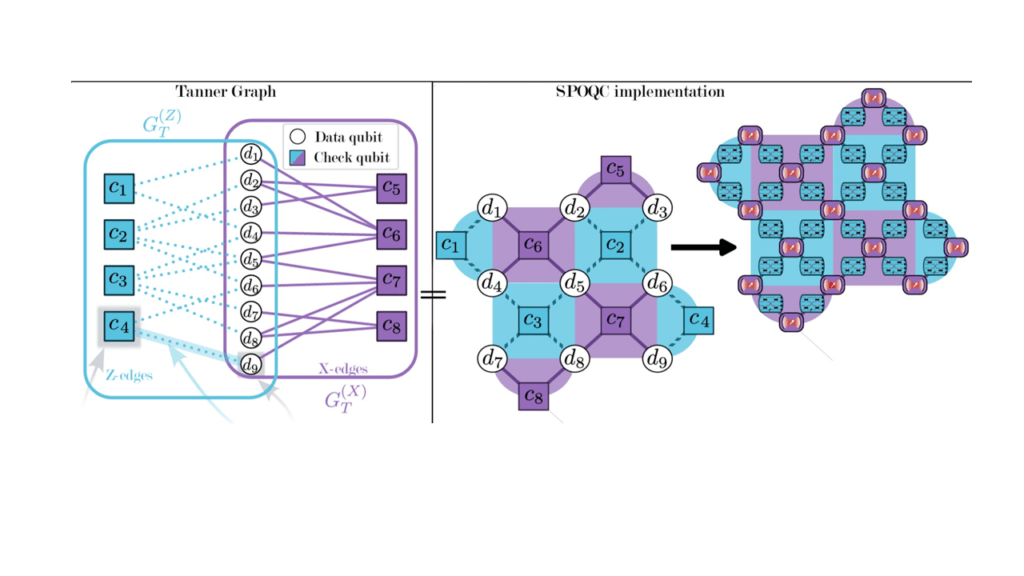
“The SPOQC architecture does not need nearest-neighbor interactions, and this is remarkably interesting since the highest performing error correcting codes actually require these long-range interactions. We can thus naturally leverage these codes to drastically reduce the resource overhead (number of components) of a fault-tolerant quantum computer, “ says Paul Hilaire, Quantum Information Scientist at Quandela.
An additional benefit from the modularity of this architecture and its use of photonic links is to isolate each module from the noise afflicting others, which is key for scalability with efficient use of qubits for error corrections.
Towards fault-tolerant quantum computers
Our SPOQC architecture enables the execution of many quantum error-correcting codes with an efficient use of qubits. Furthermore, it is compatible with many different single-photon emitters, including quantum dots, nitrogen-vacancy centers in diamonds, and rare earth ions. The successful development of the SPOQC architecture in the hardware and the implementation of the corresponding error-correcting codes will bring us closer than ever to creating a fault-tolerant quantum computer. Fault-tolerant quantum computers will be able to solve the most pressing real-world problems with the promising efficiency of quantum technologies.
References
De Gliniasty, G., Hilaire, P., Emeriau, P., Wein, S. C., Salavrakos, A., & Mansfield, S. (2024). A Spin-Optical Quantum computing architecture. Quantum, 8, 1423. https://doi.org/10.22331/q-2024-07-24-1423
Paul Hilaire, Théo Dessertaine, Boris Bourdoncle, Aurélie Denys, Grégoire de Gliniasty, Gerard Valentí-Rojas, Shane Mansfield.(2024). Enhanced Fault-tolerance in Photonic Quantum Computing: Floquet Code Outperforms Surface Code in Tailored Architecture. Available here: https://doi.org/10.48550/arXiv.2410.07065
Would you like to program a quantum computer? Register on our Quandela Cloud and start for free!